R&D Spotlight: Mesoporous Silica and poly(D,L-lactic-co-glycolic Acid) Nanoparticles for Next-generation Drug Delivery and Targeted Therapeutic Technologies
This blog post was written by Silvia Grama and Philippe Saint Cricq Riviere from Fortis Life Sciences®. Fortis partners with diagnostics and life sciences companies to design, validate, and manufacture solutions to solve their complex development problems.
Multi-functional nanoparticles are gaining attention in nanomedicine due to their unique capabilities. Nanoparticles can deliver specific compounds, target locations and destroy cells once they reach their destination. Functionalities like fluorescence, magnetism, light scattering and drug delivery can be incorporated. In many cases, biomolecules (e.g., antibodies, oligonucleotides, peptides or proteins) are bound to the particle surface to direct the particle to a specific location or target. Stealth coatings extend circulation times, helping nanoparticles evade the body’s scavenging mechanisms, and many nanoparticles can be tracked within the body to study their capabilities and behaviors in vivo.
This article highlights recent advances in the development of two unique nano-medicinal particle platforms: mesoporous silica nanoparticles (MSN) and poly (D,L-lactic-co-glycolic acid) (PLGA). MSN have high surface area and facile surface tunability, leading them to be often described as “nanosponges,” as they can adsorb large amounts of molecules dissolved in solution. PLGA is a biodegradable polymer commonly used to make particles for in vivo studies and — as silica — is generally regarded as safe. PLGA is approved by both the US Food and Drug Administration (FDA) and European Medicine Agency (EMA) for use in vaccines, drug delivery and tissue engineering.
MSN and PLGA Nanoparticles Synthesis
The synthesis procedure for both types of nanoparticles was adapted from literature and allowed for the reproducible production of large quantities of particles. The chosen method for preparing the MSN involved in-situ hydrolysis and condensation, whereas for the PLGA nanoparticles, nanoprecipitation, also known as interfacial deposition, was utilized. Both types of nanoparticles are currently used as potential prophylactic vaccines against HIV.
With an average diameter of 100 nm and enlarged pore size around 20 – 25 nm, MSN were designed to encapsulate messenger RNA coding for envelop protein of the virus (glycoprotein, gp160). To retain molecules inside the particles and prevent premature release, the silica surface was modified with pH or redox-responsive polymer capping agents. The redox-responsive polymer was based on four chains of polyethylene glycol (PEG), each terminated with a cleavable dithiol group that would react to high concentration of the reducing agent glutathione inside the targeted cells. The pH-responsive polymer was based on a small chain of polyvinylpyrridine (PVP), whose solubility would increase under the lower pH environment reached inside the late endosome due to protonation. Because of the high effectiveness of the polymer capping, lower amounts of PVP as well as lower molecular chains needed to be used to achieve appreciable encapsulation/release of cargo molecules. This mechanism also facilitated endosomal escape to the encapsulated material upon release inside the endosome. In addition, the surface has been modified to specifically target a family of professional antigen presenting cells (APC); to ensure targeting to the APC of interest (mainly dendritic cells and macrophages), mannose was grafted to the outer surface of the nanoparticles. This modification would prevent off-target delivery by making use of the DC-SIGN receptor abundantly present on the aforementioned APCs. The DC-SIGN receptor is involved in the infection of T-cells by the HIV virus, and the produced nanoparticles would then exploit the virus’s mechanism of recognition/internalization. The constructed particles were then able to encapsulate messenger RNA coding for either the green fluorescent protein (GFP) or the HIV envelope glycoprotein (gp160). These particles were able to encapsulate messenger RNAs with high efficiency, reaching 12% encapsulation by mass.
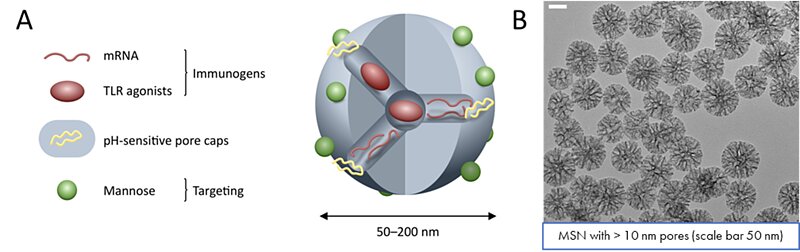
Additionally, the goal was to develop a new polymer-based nanoparticle with an optimal protein-adjuvant formulation, combining both targeting and controlled delivery for immunization against HIV.
With an average diameter of 200 nm, PLGA nanoparticles were generated through a multi-step procedure. First, the PLGA particles were loaded with another variant of the HIV envelope protein (gp140) using the nanoprecipitation method. The surface of the nanoparticles was electrostatically coated with a biocompatible polysaccharide, chitosan (CS), to introduce functionality to the system. The surface was further decorated with the same envelop protein (gp140) for an efficient multivalent uptake by the targeted B cell receptors. Besides the HIV envelope trimer (gp 140), the engineered PLGA-CS nanoparticles were also loaded with an adjuvant, monophosphoryl lipid A (MPLA), which boosted the immune response. Successful encapsulation (87%) and conjugation (45%) of the gp140 protein was detected by microBCA. The loaded PLGA nanoparticles controllably released the gp140 admixed with MPLA adjuvant to the immune system, maximizing neutralizing antibody (NAb) response due to a gradual and optimal maturation of the germinal centers (GC).
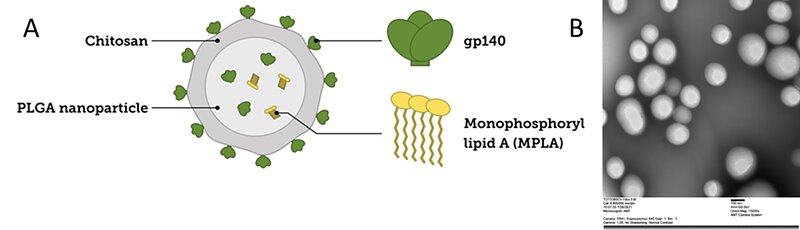
In vitro and in vivo testing
MSN in vitro: In vitro tests with bone marrow-derived dendritic cells showed that MSN nanoparticles (NPs) effectively internalized into target cells and transfected genetic material. GFP expression confirmed this after incubating nanoparticles with GFP-coding mRNA. Adding polycationic polymer polyethylenimine (PEI) improved translation, possibly due to enhanced endosomal escape, and was as a result included in final formulations for animal testing.
The PLGA NPs were not subjected to in vitro testing prior to animal testing.
MSN in vivo: The in vivo experiment was conducted using a cohort balb/c mice. The cohort was split into two main arms, a low dose (10 µg) and a high dose (50 µg) corresponding to 1 and 5 µg of mRNA injections respectively. These results demonstrated that gp160 mRNA MSN NP are poorly immunogenic in mice. However, using a preparation of gp160 mRNA + MPLA + PEI (using 4 silane 50:50 PVP:PEG MSN NP) improved immunogenicity over the other preparations and promoted the induction of low-level Tier-2 HIV-specific NAb. A variant using a different ratio of PVP:PEG, such as 1:3 instead of 1:1, might have shown better immunogenicity thanks to a better release of the genetic material as demonstrated during the in vitro optimization phase.
PLGA in vivo: NPs proved immunogenic in balb/c mice, leading to robust gp140-specific binding antibodies for various group M subtypes gp140 sequences. These levels significantly exceeded those in mice receiving the trimer alone. Additionally, mice vaccinated with the PLGA NPs developed HIV env-specific T cells comparable to mice vaccinated with the trimers only. Enhanced HIV-specific humoral response with the encapsulated, slow-release trimer compared to the non-encapsulated subunit vaccine highlighted PLGA NP’s effectiveness in generating durable and high titer HIV-specific binding antibodies.
Conclusions
Controlled MSN nanoparticles (100 nm diameter, 20 – 25 nm pores) and PLGA/CS nanoparticles were successfully synthesized and characterized. These nanoparticles can encapsulate large molecules such as mRNA or the HIV envelope glycoprotein gp160 for MSN and the gp 140 trimeric HIV envelope protein for PLGA/CS. In vitro tests using bone marrow-derived dendritic cells indicated successful internalization of MSN particles and genetic material transfection. In vivo experiments involved balb/c mice for both nanoparticles. For MSN NPs, immunization had a low response, even at higher doses. Only one variant, encapsulating mRNA, MPLA adjuvant and polyethylenimine (PEI), induced low-level Tier-2 HIV-specific neutralizing antibodies. In contrast, PLGA NPs were immunogenic in balb/c mice, resulting in robust gp140-specific binding antibodies, significantly higher than those in mice receiving the trimer alone. PLGA NPs effectively promoted durable, high-titer HIV-specific binding antibodies.
About nanoComposix
nanoComposix, a Fortis Life Sciences company, is a CDMO focused on the clinical development and manufacturing of polymer and inorganic nanoparticles. We specialize in overcoming the unique development challenges of nanomedicine therapies and nanoparticle-based diagnostics. Our expertise in nanoparticle synthesis, purification, API loading and bioconjugation expedites your development timeline cost-effectively, ensuring compliance with phase-appropriate regulatory standards. Our areas of expertise include the production of gold, silver, platinum, silica and magnetite nanoparticles with varying sizes, shapes and surface modifications to make hundreds of variants for a broad range of applications. Our dedicated nanoparticle chemists complement your R&D team, providing extensive expertise to help achieve your goals.
Nanomaterials for nanomedicine
Metallic, silica and polymeric nanoparticles are increasingly used in therapeutic research. With expertise in nanoparticle fabrication and biofunctionalization, we work with clients to develop nano-enabled cancer treatments, topical therapeutics, vaccines and more:
- Targeted delivery
- Controlled release
- Photothermal therapeutics
- Theranostics
- Gene therapy
Our CDMO services include:
- Research: Proof-of-Concept Synthesis & Characterization
- Development: Design Optimization, Scale-Up, Process Development & Validation
- Production: Transfer to & Scaled Manufacturing
Our ISO 13485:2016 and QSR-compliant facility is specifically designed for the fabrication, scale-up and analysis of nanomaterial-based devices and drugs. Cleanroom spaces are designed to accommodate projects that range from early-stage feasibility to scaled production of P1 clinical materials.
Learn about applications of nanomaterials for nanomedicine and how you can work with nanoComposix as your CDMO of choice.